
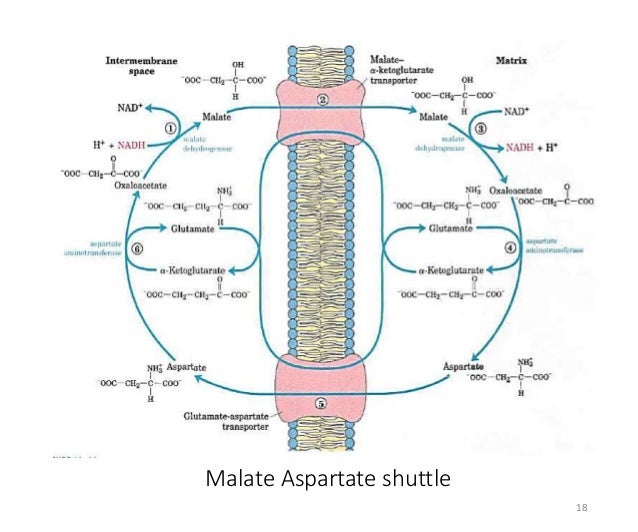
Indeed, we found that the capacity of the malate-aspartate shuttle was nearly three times greater in mitochondria isolated from neonatal than from adult porcine left ventricle ( 36). Oxidation of glucose and lactate generates cytosolic NADH, which must be oxidized for the glycolytic reactions to continue ( 26). The importance of the NADH shuttles in maintaining a continuous supply of ATP to neonatal myocardium was suggested by observations that the newborn heart relied predominantly on glucose and lactate as substrates ( 15, 25). Aspartate is then exchanged with glutamate via the aspartate/glutamate carrier (AGC) to achieve mass balance across the inner mitochondrial membrane. Oxaloacetate (OAA), formed within the mitochondrial matrix as NADH is regenerated, cannot diffuse directly across the inner mitochondrial membrane and must react with glutamate to form aspartate. 1, the reduced intermediate formed in the cytosol as part of the malate-aspartate shuttle is malate, which crosses the inner mitochondrial membrane in exchange for oxoglutarate via the oxoglutarate/malate carrier (OMC). In the heart the dominant NADH shuttle pathway is the malate-aspartate shuttle ( 33, 36), which was initially described by Borst ( 5).Īs shown in Fig. The intermediate then enters the mitochondrial matrix, where NADH is regenerated. The basis of the shuttle reactions is the reduction of a suitable cytosolic intermediate by NADH.

This finding led to the description of several “NADH shuttle” pathways, including the α-glycerophosphate, fatty acid, malate-citrate, and malate-aspartate shuttles ( 9). It was established by Lehninger ( 23) and Purvis and Lowenstein ( 30) that the inner mitochondrial membrane was impermeable to NADH. In addition, cytosolic NAD + must be regenerated to maintain maximal glycolytic flux. To achieve maximal ATP production from these substrates, NADH must reach the mitochondrial matrix to enter the electron transport chain. The glycolytic metabolism of glucose and oxidation of lactate result in the production of cytosolic NADH. It was concluded that 1) myocardial malate-aspartate shuttle capacity declines rapidly after birth, 2) divergence of mitochondrial malate dehydrogenase mRNA and protein levels during development suggests posttranscriptional regulation of this protein, and 3) the developmental decline in malate-aspartate shuttle capacity is regulated by decreased oxoglutarate/malate carrier gene expression. mRNA and protein levels of the oxoglutarate/malate carrier were increased in newborn myocardium. Aspartate/glutamate carrier protein levels were also greater in adult than in newborn tissue. mRNA levels of mitochondrial malate dehydrogenase in adult cardiac tissue were 224% of levels in newborn tissue, whereas protein levels were 54% greater in adult myocardium. Mitochondrial aspartate aminotransferase mRNA levels were greater in adult than in newborn myocardium.

By use of isolated porcine cardiac mitochondria incubated with saturating concentrations of the cytosolic components of the malate-aspartate shuttle, shuttle capacity was found to decline by ∼50% during the first 5 wk of life (from 921 ± 48 to 531 ± 53 nmol ⋅ min −1 ⋅ mg protein −1). The goals of this study were to determine the time course of the postnatal decline and to identify potential regulatory sites by measuring steady-state myocardial mRNA and protein levels of the mitochondrial proteins involved in the shuttle. Developmental downregulation of the malate-aspartate shuttle has been observed in cardiac mitochondria.
